Written by Taro Eichler, Rohde & Schwarz for 5GTechnologyWorld.com
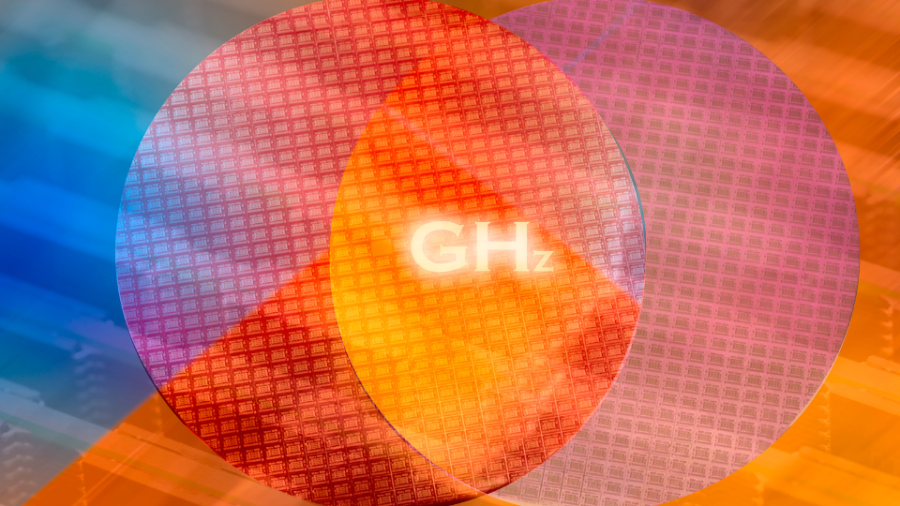
Efforts to explore and “unlock” this frequency region require an interdisciplinary approach with high-frequency RF semiconductor technology. The THz region also shows great promise for many application areas ranging from imaging to spectroscopy to sensing.
Sub-terahertz communications for 6G will become a reality only after engineers achieve a solid understanding of electromagnetic wave propagation in the as-yet insufficiently researched frequencies above 100 GHz. After achieving that knowledge, we can then derive channel models to enable system-level simulations of the new standard. Channel models for 4G and 5G cannot simply be extended above 100 GHz; engineers must verify and fine-tune knowledge to correctly reflect the impact of the environment for various use cases. We must, for example, understand outdoor scenarios and indoor industrial scenarios where human bodies, vehicles, and environmental conditions such as rain propagation strongly influence signal propagation.
5G pioneered the use of millimetre wave frequencies with bandwidths up to 400 MHz per component carrier to enable transmission rates necessary for demanding real-time applications such as wireless factory automation. 6G technology is aiming at significantly higher transmission rates and lower latencies. Large contiguous frequency ranges for ultra-high data rates with bandwidths of several GHz are only available above 100 GHz.
From channel sounding to channel models
Channel measurements by channel sounding deliver an image of the propagation properties of electromagnetic waves at a particular frequency. The term “channel sounding” comes from sonar technology, where a ship or submarine sends short acoustic pulses and records the reflections in the time domain. This provides a viable image of the surroundings.
With sonar, the transmitter and receiver are in the same place. As for channel sounding of electromagnetic waves, the transmitter and receiver are spatially separated. In time domain channel sounding, a modulated pulse signal with excellent autocorrelation properties, such as a Frank-Zadoff-Chu (FZC) sequence [1], serves as a “ping” whose channel impulse response (CIR) is recorded. This propagation-time measurement is very similar to the time-delay measurements performed in a GPS receiver in reference to the GPS satellites (and subsequently inferring the position information), where each satellite transmits its specific correlation sequence. The CIR includes both the direct propagation components (line of sight, LOS) and all reflection and scattering components (non-line-of-sight, NLOS) from objects in the environment (Figure 1). We can derive channel-model parameters and their values from the results.
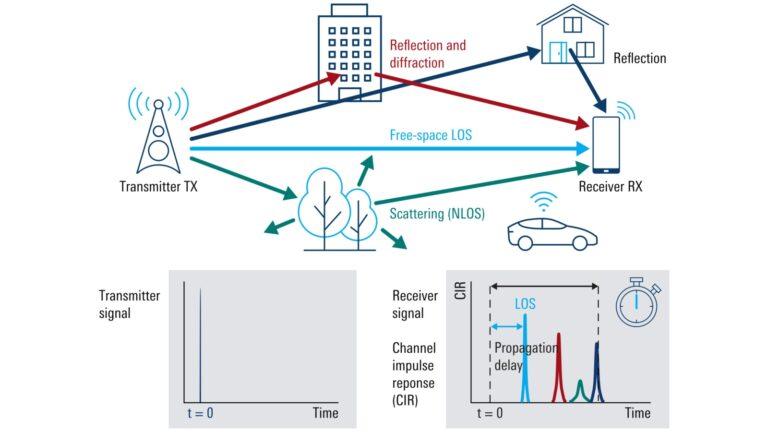
Figure 1. Operating principle of time domain channel sounding: The channel impulse response (CIR) is measured by emitting an electromagnetic “ping” at the frequency of interest and capturing all returning signal components.
Objects are only “physically visible” to electromagnetic waves and function as reflectors or scatterers when they are at least as large as the wavelength of the incident wave. This means that at a frequency of 30 GHz, objects with dimensions in the centimetre range already act as reflectors.
Time-domain channel sounding at 300 GHz
Earlier channel-sounding projects include a high-resolution channel measurement campaign at 67 GHz on a Tokyo street canyon [2]. To support the 3GPP efforts, Rohde & Schwarz collaborated with the Fraunhofer Heinrich Hertz Institute (HHI) to perform measurements in its Memmingen and Teisnach production plants, not only in the 28 GHz and 66 GHz millimetre wave frequency bands, but also in the 3.7 GHz to 3.8 GHz band designated for private campus networks in Germany [3]. Channel measurements in the Memmingen production plant have very recently been extended also to frequencies in the D-band (110 GHz to 170 GHz).
For 6G, the focus is on channels at frequencies beyond 100 GHz. In a collaborative effort with the Fraunhofer HHI and the Fraunhofer Institute for Applied Solid State Physics (IAF), we developed a research setup that performs signal generation and analysis at frequencies from 275 GHz to 325 GHz with a bandwidth of 2 GHz. The signal can be used to perform channel measurements and is modulated with novel waveforms for transmission experiments.
Several semiconductor technologies have proved relevant for mmWave applications, so 6G testing combines various semiconductor technologies to optimise performance and energy consumption. Applicable technologies include combinations of InP (speed), CMOS (integration density), and GaN (output power). In particular, InGaAs mHEMT MMICs provide for extremely low-noise and broadband applications at room temperature.
THz channel measurements
The first results for outdoor scenarios and for an indoor conference room were followed by a more systematic study of the channel propagation characteristics at sub-THz frequencies (158 GHz and 300 GHz) conducted on the campus of the Rohde & Schwarz headquarters in Munich. The study focused on two representative scenarios: a “street canyon[-like]” (urban micro-cellular UMi) scenario in a corridor between two R&D buildings [4], and an indoor measurement campaign in an atrium similar to a shopping mall or airport concourse [5].
For a “street canyon[-like]” scenario, the stationary transmitter (Tx) was placed at a height of 1.5 m using a bicycle stand. From there, the scenery leads into a more open space with a small plaza and isolated trees (Figure 2).
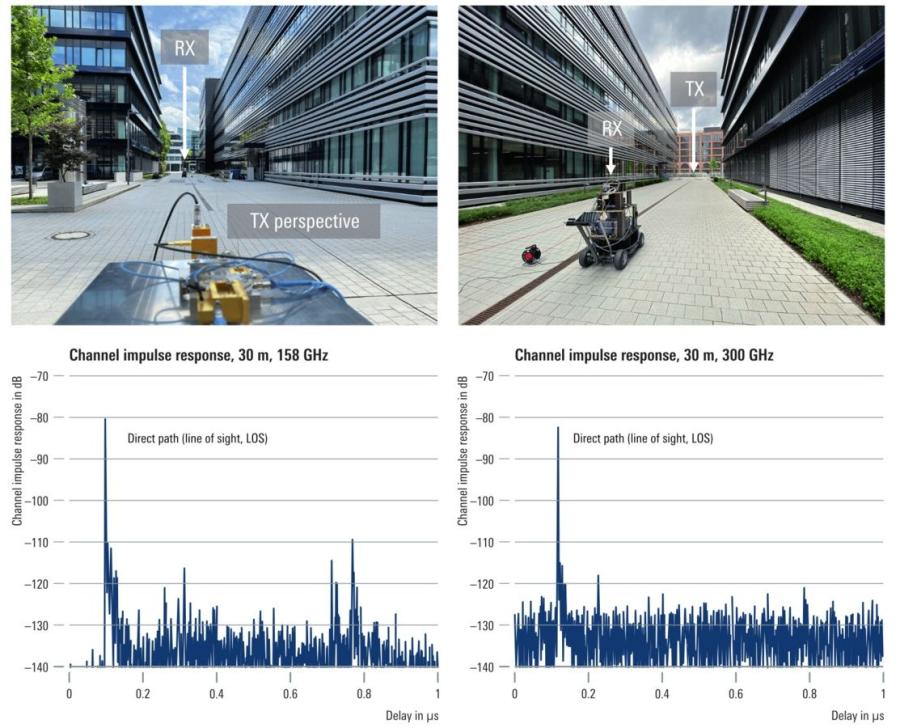
Figure 2. Engineers performed angle-resolved CIR THz channel measurements at 158 GHz and 300 GHz (D band) in an outdoor street canyon environment at Rohde & Schwarz headquarters in Munich.
We mounted the receiver (Rx) and test equipment on a wireless platform (camera dolly) at a height of 1.5 m. We positioned it at various distances from the transmitter up to 170 m. Most of the measurements were in line-of-sight. Example measurements show the CIR for aligned antennas at 30 m for 158 GHz and 300 GHz. The first peak with a delay (time of flight) of 0.1 µs represents the LOS path, corresponding to 30 m. Multipath components are also visible and more pronounced at 158 GHz than at 300 GHz. Path loss exponent can be derived from this set of large-scale measurements. We found multipath components at larger delays over nearly the complete measurement set. CIRs were measured at 158 GHz with aligned antennas over a distance from 10 m to 170 m.
Angle-resolved measurements
Further evaluation of the measured data sets involved analysing the angular information. At each measurement point, the receiver was rotated to 24 equally spaced angular positions, resulting in spatial scanning of the radio channel in the azimuth plane with 15° resolution.
Based on this estimation of paths, we can calculate the overall received power (i.e. effective overall path gain) from all paths. Although the channel at 300 GHz is much sparser than at 158 GHz, results show clearly that there is little difference in overall power. Additional paths at 158 GHz could be resolved owing to the high sensitivity of the measurement principle, but they do not significantly contribute to overall power. Furthermore, statistical parameters such as the root mean square (RMS) delay spread, and the RMS angular spread can be evaluated from these results.
Further results for the channel-measurement campaign were performed in an urban micro-cellular (UMi) street canyon scenario at 158 GHz and 300 GHz.
Indoor “shopping mall/airport” scenario
We performed indoor measurements in a building’s large open space, which acted as a shopping mall/airport scenario. The hall is around 52 m × 13 m with a ceiling height of around 20 m. The Tx antenna was configured at a fixed position in front of the elevators at the building entrance at a height of 1.5 m, whilst the receiver was mounted on a wireless platform (antenna height 1.5 m) and moved to distinct positions on a rectangular grid covering the complete floor area.
We measured angle-resolved estimated paths with the LOS peak and several multipath components (Figure 3). Indoor measurements at 158 GHz with a 40 m distance demonstrate the presence of multipath components from different directions, contributing to higher overall received power compared to the outdoor measurements (–71.4 dB at 40 m vs. –75.1 dB at 30 m).
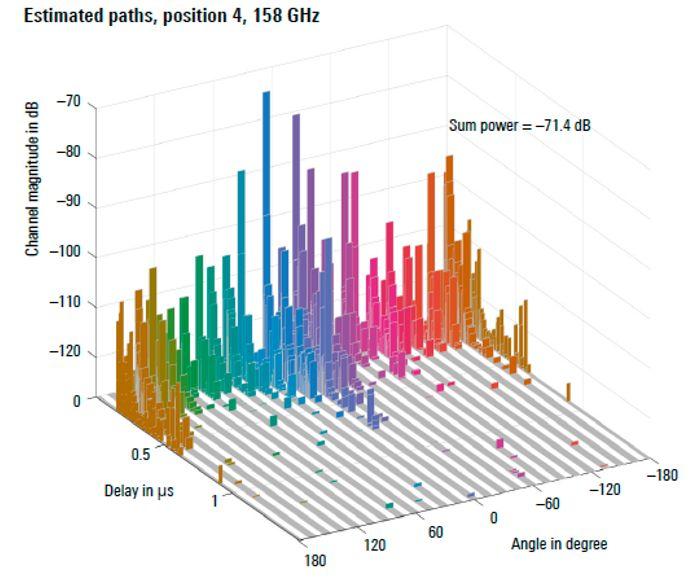
Figure 3. Estimated paths of one particular indoor position at 158 GHz for the atrium scenario (shopping mall/airport) with an angular range covering –180° to 180°
Another interesting representation of the results can be visualised using a rose plot where each “pie” represents the overall power in the respective angle bin, normalised to overall power (Figure 4). Single contributions from distinct paths within one angle bin are depicted by dots. This clearly shows that just one or two angle bins account for almost all the overall power and that within one bin, only a few paths contribute significantly. Although the test equipment received most of the power in the LOS direction, significant multipaths arrive from all measured azimuth directions.
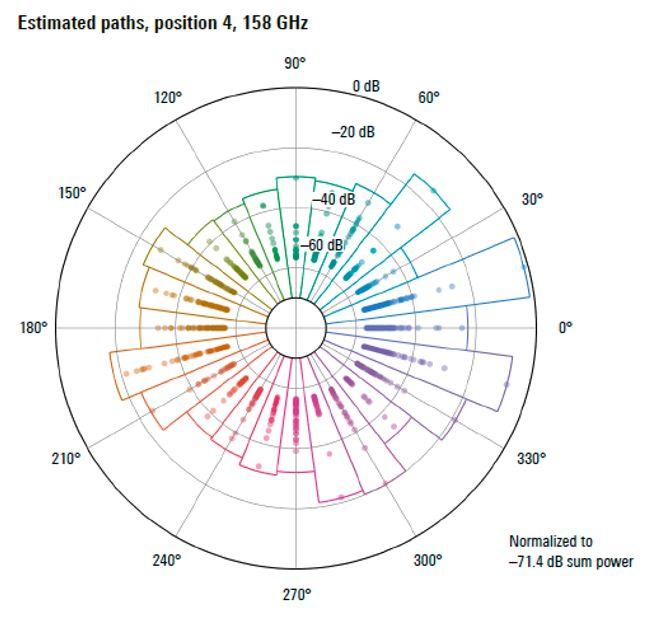
Figure 4. In the rose plot, the same data set is shown in a polar plot where each dot corresponds to one peak, and the triangle (“pie”) represents the overall power in the respective angle bin. Several multipath components appear.
We then extended the frequency range to 300 GHz and analysed angle-resolved channel-impulse responses with regards to channel parameters such as delay and angular spread. The analysis shows, with a decreasing Rx antenna directivity and an increasing SNR, the number of multipath components increases rapidly [6].
We also performed a study in the same environment at 160 GHz with a bandwidth of 4 GHz. The analysis of these extensive indoor directional channel measurements shows that with the abundance of spectrum in the sub-THz space and thus very high bandwidths, very simple modulation schemes (i.e. single-carrier) and equalisation techniques can be used while still achieving significant capacity gains. [7]
Prospects
The first results of these channel-sounding measurement campaigns have contributed to the ITU-R Working Party 5D (W5PD) report on “Technical feasibility of IMT in bands above 100 GHz” with the objective to study and provide information on the technical feasibility of mobile cellular technologies in bands above 92 GHz (IMT meaning International Mobile Telecommunications standards). The report will be consulted at the International Telecommunication Union (ITU) World Radio Conference 2023 (WRC23), where we expect additional frequency bands beyond 100 GHz to be discussed and considered for allocation at the subsequent WRC27. The current 3GPP channel model is only validated up to 100 GHz. A crucial first step for the standardisation process for 6G is to extend this channel model to higher frequencies. Future research will continue to provide insight into the fundamentals of channel propagation above 100 GHz with a focus on 6G communications.
Click here to read the original article.
References
[1] D. Chu, “Polyphase codes with good periodic correlation properties (Corresp.),” in IEEE Transactions on Information Theory, vol. 18, no. 4, pp. 531-532, July 1972, doi: 10.1109/TIT.1972.1054840
[2] M. Peter, W. Keusgen, T. Eichler, K. Yanagisawa, K. Kitao, T. Imai, M. Inomata, Y. Okumura and T. Nakamura, “High-Resolution Directional Channel Measurements at 67 GHz and Advanced Analysis of Interactions Using Geometric Information,” 2018 IEEE International Symposium on Antennas and Propagation & USNC/URSI National Radio Science Meeting, pp. 77 to 78, 2018.
[3] M. Schmieder, T. Eichler, S. Wittig, M. Peter and W. Keusgen, “Measurement and Characterization of an Indoor Industrial Environment at 3.7 and 28 GHz,” 2020 14th European Conference on Antennas and Propagation (EuCAP), Copenhagen, Denmark, 2020, pp. 1-5, doi: 10.23919/EuCAP48036.2020.9135943.
[4] W. Keusgen, A. Schultze, M. Peter and T. Eichler, “Sub-THz Channel Measurements at 158 GHz and 300 GHz in a Street Canyon Environment,” in submitted to 2022 Joint European Conference on Networks and Communications & 6G Summit (EuCNC/6G Summit), https://arxiv.org/abs/2203.04404, Grenoble, 2022.
[5] A. Schultze, W. Keusgen, M. Peter and T. Eichler, “Observations on the Angular Statistics of the Indoor Sub-THz Radio Channel at 158 GHz,” 2022 IEEE USNC-URSI Radio Science Meeting (Joint with AP-S Symposium), Denver, CO, USA, 2022, pp. 9-10, doi: 10.23919/USNC-URSI52669.2022.9887443.
[6] Alper Schultze, Ramez Askar, Michael Peter, Wilhelm Keusgen, Taro Eichler, “Angle-Resolved THz Channel Measurements at 300 GHz in a Shopping Mall Scenario,” 17th European Conference on Antennas and Propagation (EuCAP 2023), Florence, Italy, 2023.
[7] accepted for IEEE Communications Magazine (March 2023 issue), Channel Modeling and Signal Processing for Terahertz Communications, Lorenzo Miretti, Thomas Kühne, Alper Schultze, Wilhelm Keusgen, Giuseppe Caire, Michael Peter, Sławomir Sta´nczak, and Taro Eichler, “Little or No Equalization Is Needed in Energy-Efficient Sub-THz Mobile Access.”